Effect of Acetylcholinesterase Inhibitors on the Cognitive Impairments in Transgenic Drosophila Model of Alzheimer’s Disease
Abstract
Background:
Alzheimer’s disease (AD) is characterized by neuronal loss, synaptic dysfunction, deposition of amyloid-beta (Aβ), and neurofibrillary tangles (NFTs). AD patients exhibit the loss of cholinergic neurons, leads to low levels of acetylcholine and increased activity of acetylcholinesterase which further reduces the levels of acetylcholine. It has now been established that acetylcholine plays a major role in controlling cognitive functions. A number of drugs have been reported to inhibit the activity of acetylcholinesterase, which can improve cognitive dysfunction in AD patients.
Objective:
We have studied the effect of two commonly used acetylcholinesterase inhibitors (Galantamine and Rivastigmine) on the transgenic Drosophila model of AD.
Methods:
The effect of rivastigmine and galantamine was studied on cognitive parameters (Odour choice index, Open field assay, Courtship index and memory loss). Molecular docking was also performed for rivastigmine (with Aβ42 and acetylcholinesterase) and galantamine (with Aβ42 and acetylcholinesterase).
Results:
Both drugs were found to be effective in reducing cognitive defects. However, it was unclear from the data obtained which drug was more effective. The results obtained from the docking studies showed a positive interaction with Aβ42 and acetylcholinesterase for both drugs.
Conclusion:
Rivastigmine and galantamine are potent in reducing cognitive dysfunction in the transgenic AD flies expressing human Aβ42 in the neurons.
1. INTRODUCTION
Alzheimer’s disease (AD), is the most common cause of dementia. It is commonly associated with disturbed memory, thinking, and orientation [1]. Natural products can be used as drugs for the treatment of this life-threatening diseases [2]. Donepezil, rivastigmine, and galantamine are commonly prescribed drugs for the treatment of AD [3]. However, they are usually associated with numerous side effects [4-8]. Rivastigmine (carbameten) is used for the treatment of mild to moderately severe symptoms of AD. Galantamine has been reported to improve the cognitive and behavioral symptoms of AD patients [9]. Acetylcholinesterase is primarily present in blood and neural synapses, but butyrylcholinesterase is present in the liver [10]. Galantamine has been reported to exhibit therapeutic effects by its action on nicotinic receptors (nAChRs) rather than general cholinergic enhancement due to cholinesterase inhibition [11]. There is no clear evidence for the efficacy of the drugs in combating the AD symptoms [12]. To study the pathogenesis of neurodegenerative diseases, Drosophila has been established as a most successful model [13, 14]. The brain of Drosophila can be easily accessed, and various cognitive parameters can also be performed easily, making this model ideal for studying the cognitive dysfunctions associated with various neurodegenerative disorders [15]. The central nervous system of flies and humans utilizes the same neurotransmitters [14]. A number of Drosophila models are available to study the pathogenesis of AD. One such model expresses human Aβ42 under GAL4-UAS system in the brain and the flies not only exhibit diffuse amyloid deposits but also exhibit age dependent loss in the climbing ability, memory, olfaction and neuronal damage [16-18]. The effect of rivastigmine and galantamine was studied on the cognitive impairments exhibited by the transgenic Drosophila model of AD. Molecular docking was also performed to study the interactions between rivastigmine with Aβ42 and acetylcholinesterase as well as galantamine with Aβ42 and acetylcholinesterase.
2. MATERIALS AND METHODS
2.1. Drosophila Stocks, Culture and Crosses
Transgenic fly lines expressing wild-type human Abeta42 “w[1118];P{w[+mC]=UASAPP.Abeta42.B}”m26a under UAS control and GAL4“w[*];P{w [+mC]= GAL4-elavL}”3 were used in the present study (Bloomington Drosophila Stock Center, Indiana University, Bloomington, IN). The flies were cultured and crossed as described in our earlier published work [19-21]. The flies were allowed to feed cultured on standard Drosophila food containing agar, corn meal, sugar, and yeast at 25°C (24 ± 1) [20]. The AD flies were allowed to feed separately on different doses of rivastigmine mM (0.1, 1 and 10 and galantamine (0.1, 1 and 10 mM) mixed in the diet for 30 days.
2.2. Open-field Assay
Open-field Assay (OFA) was performed according to the method described by Hirth [21, 22]. The values were expressed as a mean of five independent experiments [18]. Accordingly, three flies from each group were kept in an arena divided by squares (1 cm X 1 cm) measuring 9 cm in diameter, which can be covered by a petri dish. The number of squares each fly crossed during a given time-window (30sec) was counted. The values were expressed as a mean of five independent experiments.
2.3. Courtship Assay
The assay was performed by the method of Nichols et al. [23]. Newly eclosed virgin male and female flies were separated and kept in different diet vials for 24 days at 25°C under 12 hrs light/dark. On the day of the experiment, one pair of flies were transferred into a mating chamber and observed. Observations were made until successful copulation, noting the time of each behaviour (orientation, male song, chasing and licking) and the total time of courtship behavior until mating. The courtship index (C.I.) was calculated by dividing the time of courtship by the total time until copulation [18].
2.4. Odor Choice Index
The assay was performed by the method of Simonnet et al. [24]. The test flies were starved for 16-18 hrs at 25°C before the experiment. Two small filter papers dipped in propionic acid and distilled water, respectively, were kept in the two tubes of the Y maze. After 24 days of exposure, the experiment was performed for flies of control and treated groups. The numbers of flies entering both tubes were counted. Five replicates per group and 20 flies per group were used in the assay. The odor choice index (OCI) was calculated as follows:
OCI= Number of flies in tube1- Number of flies in tube 2)/Total number of flies
2.5. Aversive Phototaxis Suppression Assay
This assay made use of positive phototactic behavior in flies to associate light with aversive stimuli (Quinine in this experiment). It was performed as described by Ali et al. [21], and data were recorded as described in our earlier published work [18]. Then, 30-day-old male flies were selected for the experiment from each group (3 replicates/group). First, the flies were trained to develop a short-term memory against 1 μM of quinine in a T-maze. Immediately after the training, the learning behavior of flies for each group was recorded as PC0 (0h postconditioning). After 6 hours, the experiment was repeated, and the readings were recorded as PC6 (6h postconditioning).
2.6. Docking Studies
The appropriate docking study between galantamine as well as rivastigmine with Aβ-42 and acetylcholinesterase was performed using HEX 8.0.0. software [25]. The structures of the drugs were drawn using CHEMSKETCH (http://www. acdlabs.com) and converted to PDB format from mol format by OPENBABEL (http://www.vcclab.org/lab/babel/). The structure of the acetylcholinesterase having PDB ID: 2ACE and Aβ42 protein fibril with PDB ID: 2ONV was obtained from the protein data bank (http://www.rcsb.org./pdb). CHIMERA (www.cgl.ucsf.edu/chimera) and PyMol (http://pymol.sourceforget.net/) molecular graphics programs were used for the visualization of the docked pose as well as the interaction between the drugs and protein/enzyme [26].
3. RESULTS
A significant decrease of 2.35-fold in the OCI was observed in AD flies compared to control flies (Fig. 1a, p<0.05). The AD flies exposed to 0.1, 1and 10 mM of rivastigmine showed a significant dose-dependent increase of 1.41, 1.52 and 1.70 folds, respectively, compared to unexposed AD flies (Fig. 1a; p<0.05). The AD flies exposed to 0.1, 1 and 10 mM of galantamine showed a dose-dependent significant increase of 1.29, 1.52, and 1.70 folds, respectively, compared to unexposed AD flies (Fig. 1a: p<0.05). A significant decrease of 6-fold was observed in the number of squares crossed by the AD flies compared to the control (Fig. 1b: p<0.05). The AD flies exposed to 0.1, 1 and 10 mM of rivastigmine showed a dose-dependent significant increase of 2, 2.46, and 3.07 folds, respectively, in the number of squares crossed compared to unexposed AD flies (Fig. 1b: p<0.05). The AD flies exposed to 0.1, 1, and 10 mM of galantamine showed a dose-dependent significant increase of 2.61, 4.15 and 5.23 folds, respectively, in the number of squares crossed compared to unexposed AD flies (Fig. 1b; p<0.05). AD flies showed a significant decrease of 1.43-fold in CI compared to control flies (Fig. 1c; p<0.05). The AD flies exposed to 0.1, 1 and 10 mM of rivastigmine showed a dose-dependent increase of 1.15, 1.27, and 1.23 folds, respectively, in CI compared to unexposed AD flies (Fig. 1c; p<0.05). The AD flies exposed to 0.1, 1, and 10 mM of galantamine showed a significant increase of 1.20, 1.26, and 1.16 folds (Fig. 1c; p<0.05). A significant decrease of 1.68-fold in memory loss was observed in AD flies compared to control flies (Fig. 1d; p<0.05). The AD flies exposed to 0.1, 1, and 10 mM of rivastigmine showed an improvement of 1.02, 1.08, and 1.20 folds compared to unexposed AD flies (Fig. 1d; p<0.05). The AD flies exposed to 0.1, 1, and 10 mM of galantamine showed an improvement of 1.12, 1.08, and 1.24 folds, respectively, compared to unexposed sAD flies (Fig. 1d; p<0.005).
The curled structure of the 2ACE is shown in (Fig. 2a (i and ii)) represents the wire form model of 2ACE, and (Fig. 2a (iii)) exhibits the surface-covered representation of the structure of the enzyme, 2ACE. Fig. (2b) illustrates the structure of the rivastigmine, which is docked with 2ACE, resulting in the docked complex. Fig. (2c) shows various docked configurations of the rivastigmine-cholinesterase interactions. The free energy of binding (FEB) of the docked model comes out to be ‒241.10 kcal mol˗1. Various non-covalent and hydrophobic interactions, as well as H-bonding interactions, play a major role in stabilizing the docked model. These interactions are depicted in Fig. (2d) in an elaborated form. The rivastigmine stabilizes itself in the docked pose via hydrophobic interactions, hydrogen bonding, and Vander Waals interactions with nearby nucleotides, as revealed in Table 1. A few such interactions are depicted in Table 1. Histidine interacts with hydrogen (HIS 406. A HD1∙∙∙ het H 0.77), carbon (HIS 406. A HD1∙∙∙ het C 1.48), and nitrogen (HIS 406.A HD1∙∙∙ het N 2.30). Asparagine, glutamine, and methionine interact with H, N and O of the drug to form H-bonds and non-covalent bonds with the enzyme. Many more such interactions are found to stabilize the drug-enzyme interactions. Fig. (2e (i)) shows the capped stick model of Aβ42, while Fig. (2e (ii)) exhibits the surface-covered model of Aβ42 respectively. Likewise, the structure of rivastigmine and its docking with the protein resulted in the docked model of the complex, depicted in (Fig. 2f), while Fig. (2g) illustrates the elaborated form of various non-covalent interactions and H-bonding in the docked model. The free energy of binding (FEB) of the docked model is ‒141.20 kcal mol˗1. Table 1 demonstrates the interactions of the different amino acid residues of Aβ42 with the drug. From the given data, it is concluded that observe valine, which is an amino acid of 2ONV, binds with the oxygen of the drug (VAL 4.A H∙∙∙ het O 1.42). Valine also makes covalent bonds with hydrogen (VAL 3.A N∙∙∙ het H 2.19) and carbon (VAL 4.A O∙∙∙ het C 2.84). Glycine makes non-covalent interactions with the hydrogen atom of the rivastigmine (GLY 2.A O∙∙∙ het H 2.44). It was observed that galantamine finds its most energetically preferred arrangement to comfortably adjust itself into the curled shape of the enzyme (2ACE).
Fig. (3a) illustrates the structure of the galantamine, which was docked with enzyme (2ACE), resulting in the docked complex, while Fig. (3b) shows various docked configurations of the galantamine-acetyl cholinesterase interactions. The free energy of binding (FEB) of the docked model was about ‒230.83 kcal mol˗1. Numerous non-covalent and hydrophobic interactions, as well as H-bonding interactions, played a major role in stabilizing the docked model. These interactions are depicted in (Fig. 3c). The drug stabilizes itself in the docked pose via hydrophobic interactions, hydrogen bonding and Vander Waals interactions with nearby nucleotides, as revealed in Table 1. A few such interactions are depicted in Table 2 below. Methionine interacts with hydrogen (MET 405.A CG∙∙∙het H 1.66) and carbon (MET 405.A CG∙∙∙het C 2.47). Histidine bonds with hydrogen (HIS 406.A HD1∙∙∙het H 1.80) and carbon (HIS 406.A HD1∙∙∙het C 1.97). Cysteine makes a bond with hydrogen (CYS 521.A SG∙∙∙het H 2.22) and oxygen (CYS 521.A CB∙∙∙het O 2.61). Asparagine, glutamine and methionine interact with H, N, and O of the drug to form H-bonds and non-covalent bonds with the enzyme. Many more such interactions are found to stabilize the drug-enzyme interactions, and a few of them are listed in Table 2. (Fig. 3d (i and ii)) shows the capped stick model and surface-covered model of Aβ42, respectively. Likewise, the structure of the Galantamine and its docking with Aβ42 results in the docked model of the complex as shown in Fig. (3e). The various non-covalent interactions, hydrophobic, and H-bonding in the docked model are shown in Fig. (3f). The free energy of binding (FEB) of the docked model was found to be ‒146.47 kcal mol˗1. Table 2 demonstrates the interactions of the different amino acid residues of Aβ42 with the galantamine. From the given table, we can see that valine which is an amino acid of 2ONV, binds with the hydrogen of the drug (VAL 3.A H∙∙∙1.het H 1.39), carbon (VAL 3.A H∙∙∙het C 2.37), nitrogen (VAL 3.A C∙∙∙1. het N 3.34) and oxygen (VAL 4.A CG1∙∙∙het O 3.79). The galantamine stabilizes itself in the docked pose via hydrophobic interactions, hydrogen bonding, and Vander Waals interactions with nearby nucleotides, as revealed in Table 2.
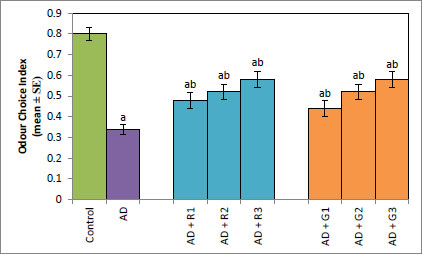
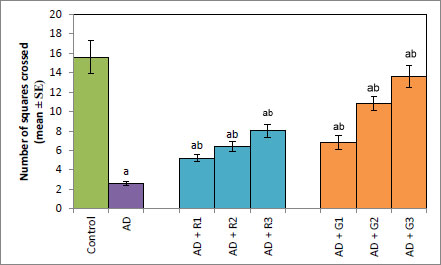
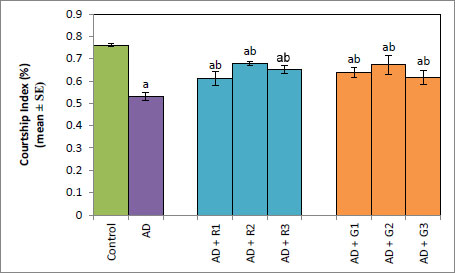
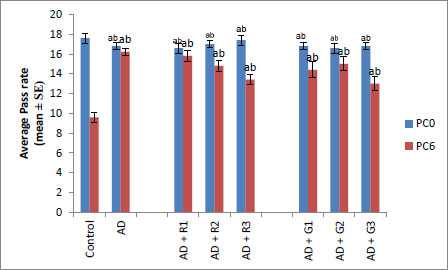
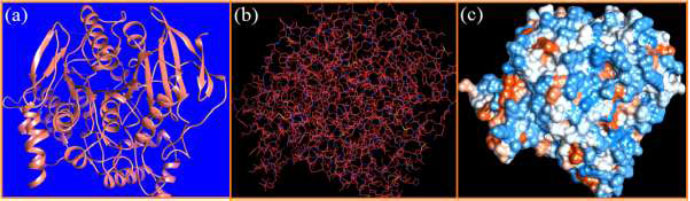
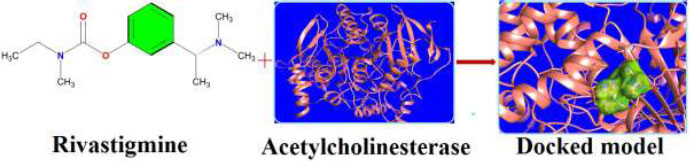
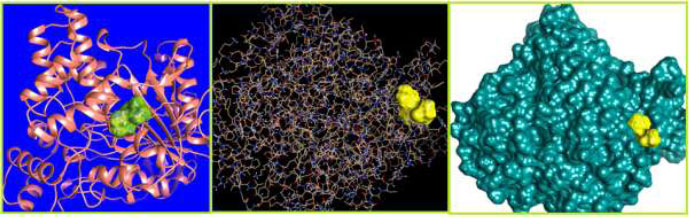
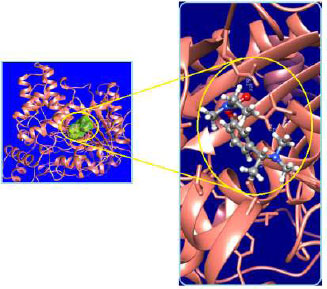
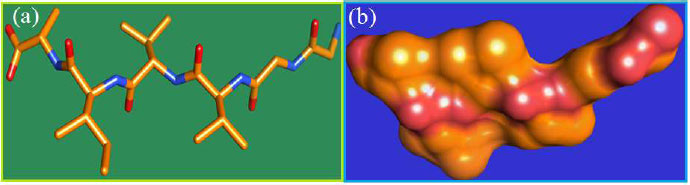

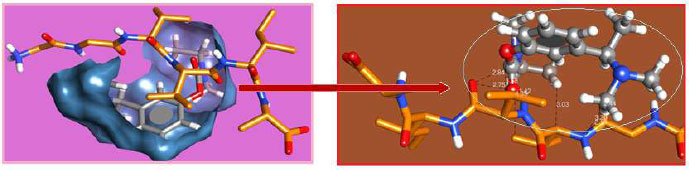
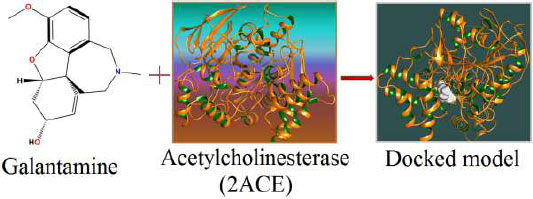
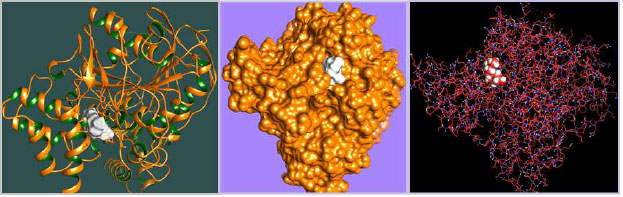
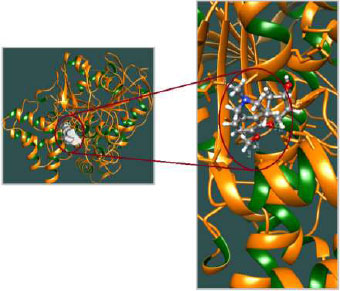
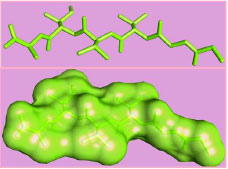
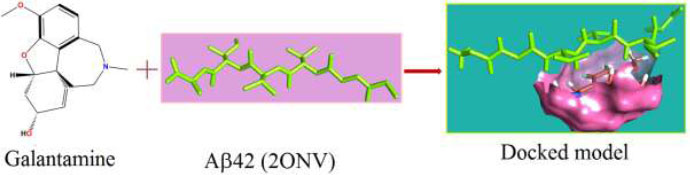
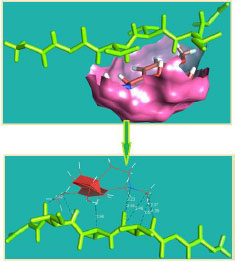
Rivastigmine+Acetylcholinesterase (2ACE) | Rivastigmine+Aβ42 (2ONV) | |||||
S. No. | ATOM 1 | ATOM 2 | Distance (Å) | ATOM 1 | ATOM 2 | Distance (Å) |
1. | HIS 406.A HD1 | 1.het H | 0.77 | VAL 4.A H | 1.het O | 1.42 |
2. | HIS 406.A HD1 | 1.het C | 1.48 | VAL 3.A N | 1.het H | 2.19 |
3. | ASN 409.A 1HD2 | 1.het H | 1.74 | GLY 2.A O | 1.het H | 2.44 |
4. | HIS 406.A HD1 | 1.het N | 2.30 | VAL 4.A O | 1.het O | 2.75 |
5. | GLN 500.A 2HE2 | 1.het O | 2.24 | VAL 4.A O | 1.het C | 2.84 |
6. | GLN 500.A NE2 | 1.het O | 2.63 | VAL 3.A CA | 1.het H | 3.03 |
7. | MET 405.A CB | 1.het H | 2.61 | VAL 3.A N | 1.het C | 3.20 |
8. | ASN 409.A 1HD2 | 1.het C | 2.72 | VAL 4.A CA | 1.het O | 3.58 |
9. | ASN 525.A 1HD2 | 1.het C | 2.93 | VAL 3.A CG1 | 1.het N | 4.35 |
10. | ASN 525.A 1HD2 | 1.het N | 3.39 | - | - | - |
Galantamine+Acetylcholinesterase (2ACE) | Galantamine+Aβ42 (2ONV) | |||||
S. No. | ATOM 1 | ATOM 2 | Distance (Å) | ATOM 1 | ATOM 2 | Distance (Å) |
1. | MET 405.A CG | 1.het H | 1.66 | VAL 3.A H | 1.het H | 1.39 |
2. | HIS 406.A HD1 | 1.het H | 1.80 | VAL 3.A O | 1.het N | 2.23 |
3. | ASN 525.A 1HD2 | 1.het O | 1.92 | VAL 3.A H | 1.het C | 2.37 |
4. | HIS 406.A HD1 | 1.het C | 1.97 | VAL 4.A CA | 1.het H | 2.96 |
5. | CYS 521.A SG | 1.het H | 2.22 | VAL 3.A C | 1.het N | 3.34 |
6. | MET 405.A CG | 1.het C | 2.47 | ILE 5.A O | 1.het H | 3.42 |
7. | CYS 521.A CB | 1.het O | 2.61 | VAL 3.A C | 1.het C | 3.49 |
8. | GLN 500.A 2HE2 | 1.het H | 2.53 | VAL 3.A CA | 1.het H | 3.57 |
9. | ASN 525.A 1HD2 | 1.het C | 2.79 | VAL 4.A CG1 | 1.het O | 3.79 |
10. | ASN 525.A 1HD2 | 1.het H | 2.83 | - | - | - |
4. DISCUSSION
The results of our present study reveal that both the drugs (rivastigmine and galantamine) are equally potent in reducing the cognitive impairments of AD flies. The olfactory organs of Drosophila consist of the antennae and the maxillary palps [27]. A variety of odors can be detected by the olfactory system of both insects and humans [28]. Neurons from the adult olfactory organs are projected directly to the antennal lobes of the brain [29]. From the antennal lobes, the projections are set towards the higher centers of the brain, i.e., mushroom bodies that control the olfactory-driven processes, such as learning and memory [30]. Neuronal cell death can be induced by the accumulation of Aβ42 [31]. The targeted expression of human disease genes in Drosophila is not only currently being used to understand the complex pathophysiology but also for the possible potential treatment of neurodegenerative disorders [32, 33]. The fly model displays a similar pathology in human AD patients, including the accumulation of Aβ containing puncta in their brains and decreased dendritic and axonal fields in the areas of the brain important for learning and memory deficits [34]. Acetylcholinesterase cleaves acetylcholine and some other choline esters. It is commonly found at neuromuscular junctions and in chemical synapses of the cholinergic type. The active site of 2ACE comprises 2 subsites—the anionic site and the esteratic subsite. The anionic subsite accommodates not only the positive quaternary amine of acetylcholine but also other cationic substrates and inhibitors. The esteratic subsite, where acetylcholine is hydrolyzed to acetate and choline, contains the catalytic triad of three amino acids: serine 200, histidine 440 and glutamate 327. Rivastigmine, works by increasing the levels of acetylcholine in the brain, which may improve the symptoms of dementia. The result of docking studies showed that the rivastigmine finds its most energetically preferred arrangement to comfortably fit itself into the curled shape of the enzyme (acetylcholinesterase). Aβ fragments are 40 or 42 amino acids long, and Aβ42 is more aggregation-prone and believed to be the toxic building block of Aβ assemblies. Aβ adopts a highly ordered structure known as the cross-β spine or amyloid [35]. Amyloid beta (Aβ or Abeta) is the main component of the amyloid plaques found in the brains of people with AD [36]. Molecular docking plays an important role in drug design and understanding the possible drug mechanism [37]. Therefore, we have docked Aβ42 fibrils and acetylcholinesterase protein fibrils with the rivastigmine and galantamine. In the case of 2ACE, H-bonding and the non-covalent and hydrophobic interactions stabilize the drug-protein docked model. Both drugs achieve for themselves, the most energetically preferred pocket to fit into a relaxed position of the protein (2ONV). Many more interactions between the galantamine and Aβ42 forming docked complexes stabilize the conformations leading to its potential application. Similarly, docking of Aβ42 protein fibril with rivastigmine attains free energy OF BINDING(FEB) for the docked model with the value -141.20 kcal mol˗1. As seen in the case of 2ACE, there exists H-bonding along with the non-covalent and hydrophobic interactions, which stabilizes the drug-protein docked model. The drug (rivastigmine) attains for itself the most energetically preferred pocket to fit into a comfortable position of the protein (2ONV). An increased risk of adverse cardiac events has been associated with using rivastigmine and galantamine [38]. Rivastigmine is more effective compared to donepezil and galantamine in reducing cognitive dysfunction [5]. The study performed on human neuroblastoma cell line SH-SYSY exposed to Aβ-42 and opadaic acid for the neuroprotective effect of donepezil, rivastigmine, and galantamine showed that donepezil, galantamine, and rivastigmine exhibit neuroprotective effect not by acetylcholinesterase inhibition but probably by the involvement of 27 nicotinic receptors and PI3K-Akt pathway [39]. Galantamine showed a protective effect against the toxicity induced by organophosphorus nerve agents in guinea pigs [40]. While comparing the anti-cholinesterase activity of donepezil, tacrine, galantamine, and rivastigmine in Dugesia tigrina, galantamine showed high anti-cholinesterase activity [41]. Galantamine hydrobromide acts both as a reversible competitive inhibitor of acetylcholinesterase (AChE) and as an allosteric modulatory of nicotinic acetylcholine receptors [42].
CONCLUSION
In our earlier study, Rivastigmine was more potent in reducing oxidative stress and improving the climbing ability of AD flies, and Galantamine was a more potent inhibitor of acetylcholinesterase, compared to rivastigmine, but Galantamine prevented the formation of Aβ-42 aggregates more effectively compared to rivastigmine [43]. Our earlier study with apigenin and nordihydroguaiaretic acid (NDGA) on the transgenic Drosophila model of Alzheimer's disease showed that the natural plant products could effectively not only reduce the oxidative stress and the formation of Aβ-42 aggregates but also improve the cognitive dysfunctions associated with the AD [44, 45].
LIST OF ABBREVIATIONS
NDGA | = Nordihydroguaiaretic Acid |
AChE | = Acetylcholinesterase |
FEB | = Free Energy of Binding |
C.I | = Courtship Index |
ETHICS APPROVAL AND CONSENT TO PARTICIPATE
Not applicable.
HUMAN AND ANIMAL RIGHTS
Not applicable.
CONSENT FOR PUBLICATION
Not applicable.
FUNDING
We are grateful to the Council of Scientific and Industrial Research (CSIR), New Delhi, India, for the sanction of the research project 37(1721)/19/EMR-II to Dr. Yasir Hasan Siddique.
CONFLICT OF INTEREST
Dr.Yasir Hasan Siddique is an Editorial Advisory Board member of the journal The Open Biology Journal.
ACKNOWLEDGEMENTS
We are thankful to the Chairman, Department of Zoology, AMU, Aligarh, for providing laboratory facilities.