All published articles of this journal are available on ScienceDirect.
Epigenetics, Maternal Diet and Metabolic Programming
Abstract
Background:
The maternal environment influences embryonic and fetal life. Nutritional deficits or excesses alter the trajectory of fetus/offspring’s development. The concept of “developmental programming” and “developmental origins of health and disease” consists of the idea that maternal diet may remodel the genome and lead to epigenetic changes. These changes are induced during early life, permanently altering the phenotype in the posterior adult stage, favoring the development of metabolic diseases such as obesity, dyslipidemia, hypertension, hyperinsulinemia, and metabolic syndrome. In this review, it is aimed to overview epigenetics, maternal diet and metabolic programming factors and determine which of these might affect future generations.
Scope and Approach:
Nutrients interfere with the epigenome by influencing the supply and use of methyl groups through DNA transmethylation and demethylation mechanisms. They also influence the remodeling of chromatin and arginine or lysine residues at the N-terminal tails of histone, thus altering miRNA expression. Fats, proteins, B vitamins and folates act as important cofactors in methylation processes. The metabolism of carbon in the methyl groups of choline, folic acid and methionine to S-Adenosyl Methionine (SAM), acts as methyl donors to methyl DNA, RNA, and proteins. B-complex vitamins are important since they act as coenzymes during this process.
Key Findings and Conclusion:
Nutrients, during pregnancy, potentially influence susceptibility to diseases in adulthood. Additionally, the deficit or excess of nutrients alter the epigenetic machinery, affecting genes and influencing the genome of the offspring and therefore, predisposing the development of chronic diseases in adults.
1. INTRODUCTION
Several environmental factors have significant effects on people's health and a great impact during embryonic and fetal development. Epidemiological studies on humans [1] and animal experiments [2] have proposed that various perinatal problems, such as placental deficiency [3], glucocorticoid exposure [4], nutritional insufficiencies or excesses [5], and stress [6], as well as maternal intestinal microbiome [7], may alter the development of the fetus/offspring, leading to a predisposition to the development of chronic non-communicable diseases. This relation between the conditions of the maternal environment and the health of the offspring leads to the concept of “developmental programming” or “Developmental Origins of Health and Disease (DOHaD)” which is defined as the response to a specific challenge to the organism in critical development that alters its course, causing health consequences that persist in adult life [8-10]. In humans, the term has been coined to refer to the first 1000 days of life, the period from conception to two years old [11]. An important finding in nutrition field was the discovery of the relation between dietary components and genome remodulation in utero and that the induction of epigenetic programming during the initial stages of life may permanently alter the organism’s phenotype in later stages [10, 12]. Substantial evidence of this theory have been found in the development of metabolic diseases, such as dyslipidemia, hypertension, hyperinsulinemia, obesity, as well as metabolic syndrome [13-15]. It has been postulated that the development of these diseases depends on an interaction between genes and risk factors in adulthood, interaction between genes and the fetal embryo, and the environment in early postnatal period [16]; therefore, the nutrition during pregnancy is crucial and may influence the health outcomes of the offspring later in life, as well as changes in nutrient composition (macro and/or micronutrients) of the maternal diet during the critical stages of fetal development [17].
Epigenetics study the heritable changes in gene expression not associated with any alteration in the Primary Deoxyribonucleic Acid (DNA) sequence, referring to the manner in which genetic information is organized, stored, and read. The modifications in epigenome include the methylation of DNA (most well-known and studied), histone modifications, the positioning of the nucleosome along the DNA and non-coding small and long Ribonucleic Acid (non-coding RNA: ncRNA), including micro RNA (miRNA) [18]. In the DNA of mammals, cytosines in Cytosine-Phosphorus-Guanine Dinucleotide (CpG islands) are frequently methylated. The chemical reaction of DNA methylation regulates the cell development, cell homeostasis and cell functions, particularly, gene expression control, repetitive DNA elements silencing, genomic imprinting, chromatin structure regulation, and the inactivation of the X chromosome in females [18]. In normal cells, the level of DNA methylation is well balanced; however, epigenetic modification patterns in individual genomic regions are affected by genetic and environmental factors [18].
Histone modifications are a complex epigenetic mechanism. This epigenetic modulation regulates chromatin structure, accessibility and transcription activities within the cell. These post-translational modifications play an important role in several cellular processes, such as gene transcription regulation, DNA repair, cell cycle, and metabolic control [19]. Recently, the importance of another epigenetic mechanism mediated by miRNAs and other small and long ncRNAs has been reported. Today, miRNAs are known to have an important role in the human genome, serving as a primary regulatory of genes encoding proteins [20]. With 16-29 nucleotides in length, this small non-coding RNA functions mainly by inhibiting the genes at the post-transcriptional level [18].
Fetal development has the characteristics of remarkable plasticity and a great capacity to react to environmental changes and lifestyle factors. Several studies have also shown that malnutrition or maternal nutrition may generate metabolic deregulation in the offspring through various epigenetic processes, such as DNA methylation, alteration in miRNA expression and histone modification, which may modulate placental gene expression and signaling during fetal development, generating dramatic changes in the programming of subsequent development [21]. In this review, it is aimed to overview epigenetics, maternal diet and metabolic programming factors and determine which of these might affect future generations.
2. EPIGENETICS
In general, nutrition conditions (e.g. high-calorie diets, calorie restriction), macronutrient derivatives (e.g., betaine, choline, and methionine), micronutrients such as vitamins (e.g., vitamins of B complex, folic acid, vitamin D, and retinoic acid), micro minerals or trace elements (e.g., Fe, Zn, and Se), and phytochemicals (e.g., polyphenols), may influence the epigenome in three ways (Fig. 1). Firstly, nutrients may remodel the structure of chromatin and lysine and/or arginine residues at the histone N-terminal tails [22]. Furthermore, nutrients directly impact the number of methyl groups for the formation of S-adenosylmethionine (AdoMet) (e.g., the supply or synthesis of methionine, homocysteine re-methylation, the supply of 5-methyltetrahydrofolate, regulation at low levels of methylenetetrahydrofolate reductase enzyme activity) [23]. In addition, nutrients modify the use of methyl groups through the mechanisms that include changes in methyltransferase activity and DNA demethylation activity [24]. Methyl-CpG binding proteins (i.e. MeCP2) have an important role in transcriptional repression due to their interaction with corepressor complexes, chromatin compaction and spatial organization of nuclear domains [25]. The aberrant functioning of methyl-CpG binding proteins is an important epigenetic pathway. Also, nutrients may alter the miRNAs expression that is implicated in the regulation of key proteins in the Folate-mediated Carbon Metabolism (FOCM) pathway [26, 27]. FOCM is involved in the regulation of homocysteine, methionine, vitamins of B complex, and methylation of proteins, histones, DNA and RNA [28].
Several dietary factors act as important cofactors of methylation reactions that interfere with the chemical availability and allosteric position of methyl groups [29]. The metabolism of carbon in the methyl groups of choline, folic acid and methionine to S-Adenosyl Methionine (SAM) acts as methyl donors to methyl DNA, RNA and proteins. Furthermore, B complex vitamins act as coenzymes in this epigenetic process [30]. The epigenetic interference of nutrition conditions, macronutrient derivatives, micronutrients, micro minerals or trace elements, and phytochemicals, is discussed in the following paragraphs.
3. NUTRITION CONDITIONS
The high-calorie diet may change DNA methylation profile. Studies performed on primates have observed that a high-calorie maternal diet may alter the chromatin structure of the fetus through epigenetic covalent histone modifications [31]. The dietary ingestion of calories was found to be significantly correlated with DNA methylation on leukocytes from 126 older individuals [32]. The study by Strakovsky, Zhang [33] showed that a high-fat maternal diet altered hepatic metabolism in the newborn in a sex-specific way. In addition, a high-fat maternal diet modulated fetal histone sirtuin 1 (SIRT1) and deacetylase protein activity in non-human primates showing that SIRT1 has an important regulatory role in fetal epigenome and metabolome [34]. Using an ovine model of maternal obesity, miRNA expression of the fetal muscle was altered showing an association with increased intramuscular adipogenesis during fetal development [35]. More recent work has observed that newborns of obese parents have altered DNA methylation patterns in printed genes [36]. In newborns, paternal obesity is associated with Insulin-like Growth Factor type 2 (IGF2) hypomethylation [37]. Environmental changes are associated with the susceptibility to sperm development. The acquired impression may be transferred to the next generation and enhance the chronic disease risk in older age [38]. On the other hand, maternal high fat diet modulates hypothalamic regulation of body weight and energy homeostasis, since it alters the gene expression of the leptin receptor, Proopiomelanocortin (POMC) and neuropeptide Y in the offspring [39]. Nutrient excess or deficit may alter the permanent deregulation of the hypothalamic circuits, including insulin and leptin resistance, which may be the basis for permanent increases in food intake and overweight [40]. These studies linked obesity with altered epigenetic regulation of several genes important for the metabolism. Maternal overnutrition is also associated with other metabolic diseases, such as hypertension, hyperlipidemia, insulin resistance and diabetes [41].
Calorie restriction without malnutrition slows aging and prevents cancer in animal models [42, 43]. Calorie restriction affects methylation and mRNAs expression patterns and chromatin structure and regulates metabolism, inflammation and lifespan [44-46]. In monkeys, moderated calorie restriction without malnutrition reduced the incidence of diabetes, cancer, cardiovascular disease, and brain atrophy [47]. In mice, calorie restriction without malnutrition prevented insulin resistance and impaired lipid metabolism in gestational diabetes offspring [48]. On the other hand, calorie restriction associated with maternal malnutrition has detrimental effects on the offspring. Research has established that low birth weight and maternal malnutrition are associated with insulin resistance in the key organs that control glucose homeostasis, establishing a direct association between maternal undernutrition and altered pancreatic β-cell function with the development of type 2 diabetes mellitus [49]. The possible involvement of deregulation in microRNA expression in the endocrine pancreas has been hypothesized as a mediator of early nutrition in the formation of β-cell mass and the subsequent risk of diabetes mellitus type 2 [50]. Recently, it has been discovered that rodents that were exposed to maternal undernutrition and subjected to a low dietary protein content during gestation, showed a decrease in pancreas and β-cell mass, as well as impaired function in fetal development. MicroRNA profiles have revealed an increased expression of different microRNAs, including miR-375, in the pancreas of the fetuses of mothers consuming a low-protein diet; this miR-375 is specifically expressed in the islet cells of the pancreas necessary for normal pancreatic genesis, influencing not only the β-cell mass but also insulin secretion [51].
4. MACRONUTRIENTS
As for lipids, recent studies have shown that the inadequate intake of long-chain polyunsaturated fatty acids (LCPUFA) during pregnancy may provoke aberrant DNA methylation patterns affecting clinically relevant gene expression, such as angiogenic factor genes [52, 53]. This may not only contribute to vascular deregulation associated with abnormal placentation but may also have harmful effects on fetal programming, resulting in an enhanced risk of cardiovascular disease in adulthood [54]. Specific components of the Mediterranean diet assessed by the PREDIMED study, specifically omega-3 fatty acids from extra-virgin olive oil and nuts, induce methylation changes in peripheral white blood cells associated with intermediate metabolism, diabetes, inflammation and signal transduction [55]. In another study performed on humans, prenatal olive oil intake altered placental histone acetylation of immune regulatory genes [56]. In mice, the diet enriched with LCPUFA omega-3 produced epigenetic changes in the promoter of leptin [57]. Additionally, in animal models, the diet supplementation with fish oil is associated with lower DNA methylation in the liver which persists for two generations, decreased lipid concentrations in blood, increased insulin-stimulated glucose uptake, and insulin sensitivity [58].
Other important nutrients during fetal development are amino acids. The metabolism of amino acids, such as glycine, histidine, methionine and serine, have a key role in the supply of methyl donors for the synthesis of DNA and proteins. For example, a diet low in proteins is associated with the deterioration of fetal growth and the development of obesity, hypertension and diabetes in the offspring [59]. Another example is the fact that protein restriction in the prenatal stage changes the extent of DNA methylation and the expression of the angiotensin 1 receptor gene (Agtr1), which has a role in the development of hypertension [60, 61]. It has also been reported that a maternal diet low in protein has an effect on the methylation status of the promoter gene and the expression of the glucocorticoid receptor gene (Nr3c1) and the Peroxisome Proliferator-activated Receptor (PPAR) in the liver through the acetylation of histones, H3 and H4, and the methylation of lysine H3K4. Both Nr3c1 and PPAR has an important role in normal embryogenesis and the regulation of lipids or blood pressure in adulthood [40, 62].
Carbohydrates also have an important epigenetic role [63]. For example, it has been observed that high glucose levels link diabetes and cancer via epigenetic alterations [64]. Hyperglycaemia also induced epigenetic changes in the promoter of nuclear factor kappa B (NF-κB) subunit p65 in aortic endothelial cells [65].
5. MICROMINERALS OR TRACE ELEMENTS
In the case of vitamins and minerals, studies conducted on mice showed that mice born from mothers administered with an excess of folic acid and other nutrients, such as vitamin B12, methionine, Zn, betaine and choline, have an altered epigenome [66, 67]. Choline has an active role in the epigenetic mechanisms, i.e., optimal intake of choline is involved in the transference of carbon or in methylation pathways, such as folic acid, since it helps in achieving fetal development successfully. Studies on animals showed that maternal choline supplementation during pregnancy modified histone and the methylation of DNA in the fetal liver and brain [67, 68].
A study on vitamins showed that the increase in the maternal levels of vitamin B12 during pregnancy was related to a decrease of DNA methylation in newborns, while the increase in serum levels of B12 was related to the decrease of IGFBPC3 gene methylation in intrauterine growth [69]. Another example of the studies on vitamins is the case of folic acid, a known donor of only one carbon for the methylation and synthesis of DNA [70]. In early postnatal development, when cell growth and proliferation processes are crucial, folic acid is a key factor [71].
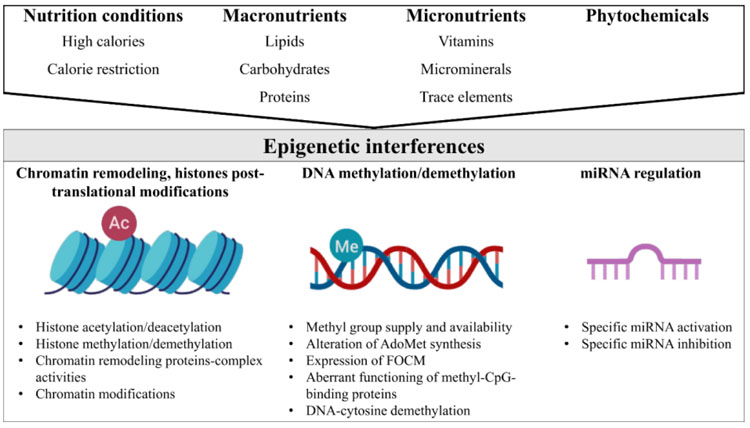
With the progression of pregnancy, folate is required in order to ensure the correct fetal development and placental functions. Folate prevents neural tube defects during fetal development [72] and its deficiency is associated with severe neurodevelopmental and neurological disorders [73]. Fryer, Nafee [74] observed that the intermediate levels associated with folate in the blood of the umbilical cord during the final stage of fetal development are inversely associated with the methylation of repetitive LINE-1 elements in lymphocytes from the umbilical cord of mothers who daily intake folic acid supplements. These results support the fact that folate and other intermediate carbon compounds have a regulatory role through the epigenetic mechanism of methylation of DNA. Restricted intake of methyl donors during the periconceptional period produces adverse phenotypes in the offspring of sheep, which has been correlated with the alterations in the DNA methylation of approximately 4% of the CpG islands. These results suggest that the epigenetic alteration of a small subgroup of genes is crucial for the programming events [74].
However, in the deficiency of folate,, methionine and vitamin B in pregnancy produces an alteration in the methylation of DNA, insulin resistance, an increase in high blood pressure, gene expression and changes in methylation related to the renin-angiotensin system, mitochondrial metabolism and phospholipid homeostasis [75]. Otherwise for vitamin B12 and its interaction with folate, a study showed that children whose mothers had low levels of vitamin B12 and high levels of folic acid were more insulin resistant and had a higher body fat percentage and abdominal fat at 6 years of age, which increases the risk of developing diabetes type 2 in the future [76]. Additionally, deficiencies of folic acid and vitamin B12 during pregnancy and breastfeeding cause signs of fetal programming, such as a decrease in weight at birth, an increase in central fat mass, liver steatosis and myocardial hypertrophy [77, 78]. The underlying molecular mechanisms are related to a decrease in the activity and expression of SIRT1 and PRMT1 and the posterior hypomethylation and hyperacetylation of PGC1-α [78].
Another nutrient mentioned is Zn, which is needed for the expression of numerous genes [79]. Due to its role in DNA methylation, Zn levels may have an essential role in the epigenome. It particularly plays a role during intrauterine life and childhood and its deficiency may contribute to an alteration in promoter methylation, which results in immune deregulation that may increase the future development of chronic diseases [80]. Thus, the results obtained in the studies carried out on animals showed that maternal Zn supplementation is associated with lower levels of DNA methylation in intestinal cells, which may alter the inflammatory status of intestinal mucus [81].
6. PHYTOCHEMICALS
Other nutrients related to epigenetic mechanisms are phytochemicals, such as lycopene, genistein, resveratrol, sulforaphane, epigallocatechin-3-gallate (EGCG), curcumin and allyl sulfur compounds [82-84]. However, the effects of some of these phytochemicals on epigenetic mechanisms are not elucidated well. They may act as demethylation agents that inhibit the methylation of DNA catalyzed by DNA (cytosine-5)-methyltransferase (DNMT1) in a dosage-dependant manner, predominantly through a non-competitive mechanism [18]. Besides, studies have shown that resveratrol reverted epigenetic and transcription changes induced by cigarette smoke in rats [85]. Maternal resveratrol supplementation prevented cognitive impairment in mice offspring through epigenetic changes and cell signaling pathways [86]. In another study performed on rats, maternal green tea polyphenol intake during lactation attenuated tubulointerstitial fibrosis and macrophage infiltration by down-regulating epigenetic modulators, such as DNMT1 [87]. Also, cannabis exposure during sensitive periods of development may alter gene regulation via epigenetic pathways [88].
6.1. Gut Microbiota and Metabolic Programming
Gut microbiota has a key role in obesity, which broadens the relationship between the composition of gut microbiota and nutritional status of the host, immune system and vulnerability to numerous diseases [89]. Also, it is established that the formation of gut microbiota starts before birth under intrauterine environment [90]. Thus, several studies have provided evidence to support that supplementing with prebiotics and probiotics, specifically during pregnancy, modifies the methylation status of DNA, particularly of obesity promoters and genes related to weight gain in mothers and their infants [91, 92]. Some examples of maternal prebiotic during pregnancy associated with programming offspring health, in animal models, are short-chain fructo-oligosaccharides that improve gut defense and immune response [93], galacto-oligosaccharides and inulin mixture that prevent food allergies [94] and oligofructose that decreases the risk of obesity [95]. Vahamiko, Laiho [96] carried out a pilot study that aimed to find out whether the administration of probiotic supplements during pregnancy might change DNA and the methylation status of obesity promoters and the genes related to weight gain in mothers and their children. The methylation status of DNA was analysed for obesity genes (623 genes) and the promoters of the gene related to weight gain (433) in children and women. The results suggested that supplementing with probiotics led to lower levels of methylation of DNA in 37 gene promoters and, in women, an increase in the levels of DNA methylation of a gene promoter. In their children, 68 gene promoters were altered, with the group supplemented with probiotics having a lower level of DNA methylation [96]. Wickens, Barthow [97] showed that early probiotic supplementation in pregnant women with a history of atopic disease reduced gestational diabetes mellitus. In another study, probiotic supplementation significantly reduced gestational weight gain and fasting blood glucose and improved insulin sensitivity [98]. In pregnant women, the consumption of probiotic yoghurt has shown beneficial effects for the mother and child [99, 100].
CONCLUSION
To conclude, nutrients and supplements may modulate epigenetic processes and metabolic programming during certain crucial moments of the fetal and early postnatal development. Identifying epigenetic processes altered by nutritional changes may help in finding new strategies to address the current problem in treating chronic diseases.
As seen in this review, the maternal diet might have a lifetime effect on the genome of a child and may potentially influence the risk of developing complex diseases in the posterior adult stage. Several components of the diet of the mother can alter epigenetic prints on the DNA. The specific effect of each type of dietary epigenetic modulator or their combination on the life of a fetus is still questioned due to insufficient data and methodological limitations.
One of the main characteristics of epigenetic mechanisms is their ability to respond to modulation, which makes early nutritional intervention possible in order to modify the risk of illness that may present in the long-term. Nonetheless, this modification may only be possible in specific epigenetic changes, which makes the identification of such changes a matter of great importance. This way, it would be easier to improve the environment that the fetus/offspring is exposed to during the critical moments of its development, which may be an important effort in public health to prevent long-term illnesses. There are three important topics to be highlighted, the effects on fetal programming when dietary factors are in excess or deficient amount, need to be better understood, the effect of the “dietary epigenetic modulators” on an organism genetically predisposed to specific illness, including transgenerational effects, and finally, the combined effect of different dietary epigenetic modulators or chronic exposure to a diet.
Future research is needed to better understand the impact of nutritional programming on the early life and its effect on biological functions. Also, research on epigenome modulators considering individual polymorphism is still scarce. Research on the impact of the cooking methods or industrial treatment on dietary epigenetic modulators present in food is also required. Investigating into this might open a new field of nutritional-epigenetic study that will contribute to guiding public policies that will determine the optimal dosage levels rather than minimal ones in order to satisfy the needs of both the mother and the fetus and benefit their health. Public policies should not only focus on the health of the population but also on the genetic printing of future generations that will be the next users of the health system.
CONSENT FOR PUBLICATION
Not applicable.
FUNDING
None.
CONFLICT OF INTEREST
The authors declare no conflict of interest, financial or otherwise.
ACKNOWLEDGEMENTS
The authors would like to thank the translators Alejandra Carrillo and Catalina Gómez.